11/23/2022
| Dr. Lisa Wild
In this article, we will tell you something about the parameter dissolved oxygen in general and the different measuring methods. After reading this you will be able to choose the right sensor for your application.
Sometimes there are just too many choices, and it is hard to decide which product is the right for your application. Dissolved Oxygen is a very important parameter in many applications such as Dinking Water and Wastewater Treatment, Aquaculture, Industry as well as Environmental Monitoring. We mainly distinguish between two measurement methods, which include the amperometric or electrochemical and the optical method. Both methods have a reason to exist, but for different applications and concentration ranges. In this article, we will explain the fundamentals of dissolved oxygen and discuss the advantages and disadvantages of both measurement methods in water or liquid media.
The electrochemical determination of dissolved oxygen in liquid media
The bases for the electrochemical determination of oxygen concentration are membrane-covered electrochemical sensors. [1] The main components of the sensor are the oxygen-permeable membrane, the working electrode, the counter electrode, the electrolyte solution and potentially a reference electrode (Fig 2). Between the gold cathode and anode, which is made from either lead or silver, there is a voltage that causes the oxygen to react electrochemically. The higher the concentration of oxygen is, the higher is the resulting current. The measured parameter is the current in the sensor, which can be converted into the concentration of the dissolved oxygen following a calibration. If the anode is made from silver, the meter supplies the required voltage (amperometric sensor). If the anode is made from lead, it is a self-polarizing sensor i.e. the voltage is created by the two electrodes in the sensor itself in the same way as a battery (galvanic sensor). The measuring meter only evaluates the current. In the case of the electrochemical determination of oxygen the following electrode reactions take place (Fig. 2).
Oxgen is reduced at the cathode:
O2 + 2 H2O + 4 e- → 4 OH-
Here “the cathode supplies electrons” and the oxygen which has diffused through the membrane reacts with the water hydroxide ions.
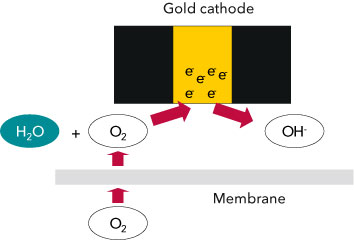
Figure 2. The principle of electrode reactions.
The electrode metal is oxidized at the anode, whereby the electrons required for the cathode reaction are set free.
The reactions that take place are either:
Ag → Ag+ + e-
or
Pb → Pb2+ + 2 e-
The equations of the anode reactions highlight the effect of the electrolyte solution. The components of the electrolyte solution bind the metal ions that result from the anode reactions. The electrolyte solutions must be suitable for the type of electrode. Amperometric oxygen sensors such as the TriOxmatic® require an ELY/N solution.
Ag+ + Br- → AgBr ↓
or
Pb2+ + 2 OH- → 2 Pb(OH)2 ↓
Pb(OH)2 → PbO + H2O
The resulting, sparingly soluble substances also prevent the lead or silver coating, fouling is a biological coating of the gold cathode which would take place if the ions were not captured.
Amperometric sensors can be operated as a three-electrode cell with an additional silver/silver bromide electrode. They no longer have any anode in the traditional sense. One of the silver/silver bromide electrodes takes on the task of the counter electrode (current dissipation), and the other the task of an independent reference electrode. This is current less and shows a fundamentally better potential constancy than a conventional electrode.
The optical determination of dissolved oxygen in liquid media
A more recent technology for the determination of dissolved oxygen is the optical measurement. [2] A sensor for optical measurement is also described as an optode. Optodes equipped with special dyes show optical measurable reactions when they come in contact with the specific molecules. A mass and energy conversion does not take place as for example with the Clark cells. It is only an energy conversion because an incident light beam of a certain wavelength is changed into light of longer wavelength and other properties than the original light. This type of reaction is known as fluorescence (Fig. 3). As part of this, dye molecules are excited by light. Upon return to the ground state the absorbed energy is emitted in the form of light with changed (larger) wavelengths. There are substances which measurably affect this mechanism depending on their concentration. These are the so-called quenchers. This means that these materials absorb the energy of the excited state so that the dye can no longer emit fluorescent light and is thereby “extinguished”. The intensity of the fluorescent light becomes smaller the higher the concentration of the quencher molecule is.
This context is basically described in the Stern-Volmer Equation:
Here I0 is the light intensity without the quencher, I the intensity with the quencher in a corresponding concentration, kSV the Stern-Volmer constant and cQ the concentration of the quencher. In this context is also interesting that not only the intensity but also the temporal decay of the fluorescent light after excitation continues to behave correspondingly with the Stern-Volmer Equation.
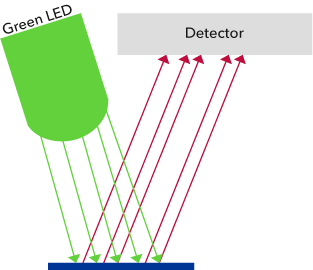
Figure 3. Luminescence excitation using light of a short wavelength (green here),
emission of a red and so low-energy fluorescent beam.
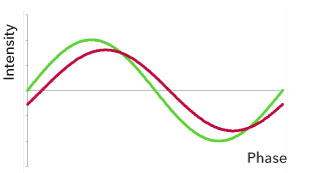
Figure 4. Simplified representation of modulated stimulus and emitted fluorescence beam.
What does this mean for the dissolved oxygen measurement? There are dyes which are excited by visible light and react on oxygen with a high selectivity. The oxygen molecules serve as the quenchers described above. They extinguish or change the light resulting from the dye depending on the oxygen partial pressure. By means of this dye suitable membranes can be produced for the measurement of oxygen in fluids or gases.
LEDs serve as the light source. The exciting of the fluorescence takes place in a modulated manner (Fig. 4). The light emitted from the dye in the membrane is detected, converted into an electrical signal, and converted into an oxygen signal.
Green graph: light periodically beamed in.
Red graph: light emitted from the dye at a shifted phase resulting from the changed decay ratio.
Advantages of optical sensors over electrochemical sensors
The significant difference from the amperometric dissolved oxygen sensors is that optical sensors consume no oxygen. There are no electrochemical reactions. In addition, there is a further characteristic: The optical sensor does not require any flow to replace “consumed” oxygen. Stirring systems are then superfluous. In addition, there is no necessity for the electrolyte change or cleaning the electrode system. The life span of the FDO® 700 IQ (link) caps with our Green-light Technology, which avoids the bleaching of the fluorescent dye in the sensor membrane, is at least one year but can range from 3 to 5 years.
Electrochemical sensors form in water a 10 µm thick layer of water molecules that cannot be stirred. As consequence this layer serves as an additional diffusion barrier. On air these sensors show a saturation signal of about 102 %. The optical sensor measures 100 % air saturation as this barrier does not exist here.
However, it may be reasonable to apply flow to an amperometric sensor. As with all systems with interfaces, even when using the optical dissolved oxygen sensor, there is diffusion of the dissolved oxygen in the water depending on the concentration into or out of the membrane. This process naturally runs faster the better the exchange takes place at the interface. A stirring supports this exchange.
There is no polarization time for optical sensors as it has no electrodes which must reach an operating condition.
Optical dissolved oxygen sensors are sensors which have active components (LEDs, detector, processor). In contrast to the electro-chemical sensors, they are more power consuming which leads to a markedly reduced operating time in the case of portable systems.
Moreover, the measurement range is noticeably restricted in comparison to the electrochemical sensors (up to 60 mg/l) above. The reason for this is the quenching. Simplified, no oxygen leads to a strong signal, a large amount of oxygen leads to a weak signal. In general, the measurement range does not exceed approx. 200 % air-saturation respectively 20 mg/l.
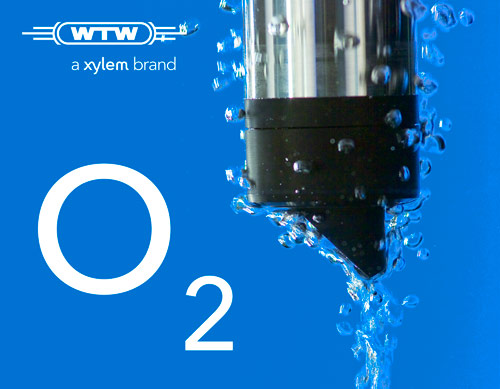
Figure 5. Air bubbles flow around the FDO® 700 IQ.
An advantage of the FDO® 700 IQ sensors is the very stable drift behavior. They are factory calibrated and do not require a calibration unlike the TriOxmatic® 700.
In contrast to the amperometric sensors, carbon dioxide and hydrogen sulfide play no role in relation to the operation life of or damage to the optical sensors. It must solely be noted that, for example, hydrogen sulfide also functions as a quencher due to its molecular mass. An example from practice would be the decreasing oxygen content in limnological depth measurements at increasing depths, which seem to undergo an increase if the sensor is immersed into soft sediment. However, the reason for this is the hydrogen sulfide, which results from the putrefaction processes in many waters, that here dissembles an oxygen signal. This signal is reversible, the sensor does not undergo any damage.
Optical Oxygen sensor can be influenced by air bubbles in the media; however, this influence has been eliminated with our 45° technology for the FDO® sensors (see Fig. 5). The membrane has a horizontal slope of 45° and avoids congestion of air bubbles in front of the membrane. In addition to being calibration and flow-free, they are therefore also insensitive to air bubbles and do not require any additional installation equipment.
Maintenance and Cleaning of the two different sensor types
The sensitive surface of an optical oxygen sensor is protected by a light proof top layer. This top layer is very thin and must therefore not be scratched or damaged as otherwise this could lead to malfunctions. The membrane is for both sensors the part which is the most sensitive to contamination. In the case of easily adhesive contamination, it is recommended to carefully clean the membrane with a soft microcloth. Deposits of calcium carbonate or iron oxide can be dissolved with 5 to 10 % (weight percentage!) concentrated acetic or citric acid and for grease and oil, warm (<50 °C) tap water with dish washing agent can be applied. Further notes can be found in our operating instructions.
How to maintain the optical oxygen sensor such as WTW FDO® 700 IQ?
As mentioned above the optical oxygen sensors have a very stable drift behavior and changes irradiated light are generally much smaller than 5 % of the initial value over the course of a year. If this tolerance can be accepted, the sensor does not need to be calibrated.
The caps of the FDO® 700 IQ need to be exchanged every 3 to 5 years. We apply dye to changing caps that the user simply puts onto the sensor. These exchange caps contain a chip with the specifications of the individual membrane determined in the production process (“factory calibration”). This data is automatically transferred to the sensor and used for the correct calculation of the oxygen concentration. Of course, a calibration carried out by the user is also possible. It is implemented analogously to the electrochemical sensors in water-vapor-saturated air in the calibration vessel. As already mentioned above, the resulting saturation display is at 100 %.
How to maintain the electrochemical oxygen sensor such as WTW TriOxmatic® 700 IQ?
The electrochemical sensors require an exchange of the membrane and the electrolyte twice a year or depending on the application. Moreover, the gold cathode of the electrochemical sensor should be examined for a visual check. If it is no longer gold in color, but instead covered with lead or silver, the sensor shows high values and is generally no longer free of a zero-current. A remedy to this can be found through regenerating the dissolved oxygen sensor according to the operating instructions. The gold cathode should be polished only using a special, moistened polishing strip with circular movements and little pressure. Only this strip must be used in any circumstances as a scratched and unpolished electrode surface damages the sensor and negatively affects the measuring reliability.
The optical sensor (
FDO® 700 IQ) requires no calibration as it comes factory calibrated and stays stable for the lifetime of the cap (at least one year). However, a calibration can be performed if required for regulatory compliance or quality assurance. The electrochemical sensor (
TriOxmatic® 700 IQ) should be calibrated twice a year or depending on the application. For both sensors, calibration takes place in water vapor-saturated air. To do so, the sensor should be positioned approx. 2 cm above a water surface, for example in a narrow bucket or similar container with water. In the case of air temperatures under 5 °C we recommend calibrating not in air but in air-saturated water that has a higher temperature. You obtain air-saturated water by pouring water several times in and out of two vessels so that it sparkles.
In conclusion it can be said that the optical sensors offer mainly advantages over the amperometric sensors. The fact that the optical FDO® sensors are flow-free, insensitive to air bubbles and require no calibration, they are the optimal sensor to measure dissolved oxygen in wastewater, surface water, drinking water and aquaculture applications at a measuring range of up to 20 mg/l. However, if there is a need to measure processes with dissolved oxygen concentrations of up to 60 mg/l or 600 %, our amperometric sensor the TriOxmatic® 700 IQ is a better choice.
If you need further information, you will find application reports on optical or amperometric sensors below, as well as on the validation of oxygen sensors here as a download.
However, if there is a need to measure processes with dissolved oxygen concentrations of up to 60 mg/l or 600 %, our amperometric sensor the TriOxmatic® 700 IQ is a better choice.